Positron emission particle tracking (PEPT) is a technique used to non-invasively visualise and measure parameters of flow in wet, dry or multiphase systems. PEPT relies on the 3D tracking of a radiolabelled tracer particle used as a flow follower within the field of view of a modified PET scanner, at speeds approaching 10 m/s with location uncertainties smaller than 1 mm.
It is derived from the medical technique positron emission tomography (PET), repurposing its detector hardware, the positron camera or PET scanner, and tracer techniques for science and engineering applications. It can track single or multiple tracer particles inside experimental vessels that fit within the field of view of the PET camera.
PEPT was developed in the early 1990s by a group at the University of Birmingham. After the Positron Imaging Centre at the University of Birmingham, PEPT Cape Town became the second operational PEPT facility in the world during 2009. Over the last decade, a wide range of PEPT applications have been explored, in particular, powder mixing, particle and fluid behaviour in granular beds, rotating drums, stirred tanks and flotation cells.
From a physics perspective, a tracer particle is pre-labelled with a proton-rich nuclei which decays via positron emission. After annihilation with an electron (from surrounding matter), a pair of nearly co-linear photons is released.
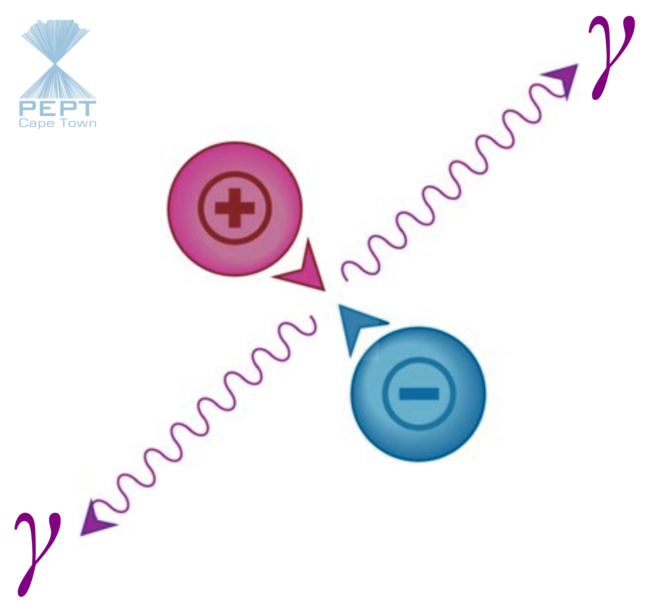
Positron-electron annihilation producing almost back-to-back photons with energy 511 keV.
The PET scanner or positron camera such as the Siemens HR++ at PEPT Cape Town is illustrated here and contains an array of detector elements under the cover.
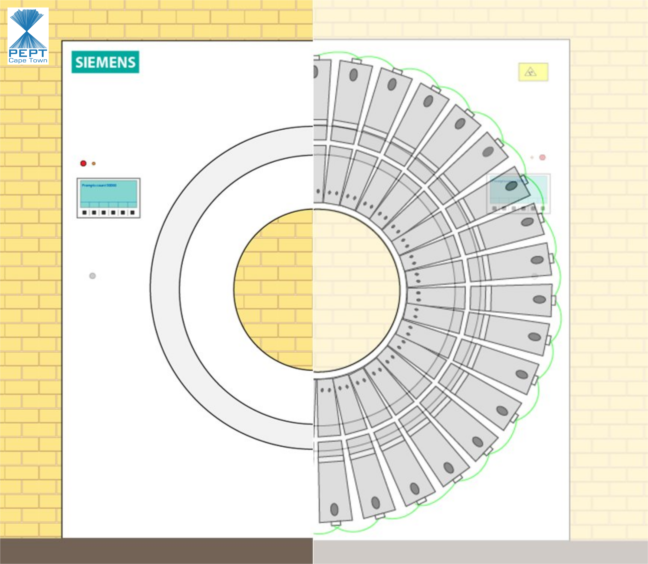
Cutaway illustration of the HR++ camera detector geometry.
If both of these 511 keV gamma rays are detected in coincidence by two of the detectors within the positron camera, a line of response (LOR) can be defined. This LOR defines a path along which the annihilation occurred, which is approximately the positron of the tracer particle. With two such LORs, the location of the tracer can be triangulated within three dimensions.
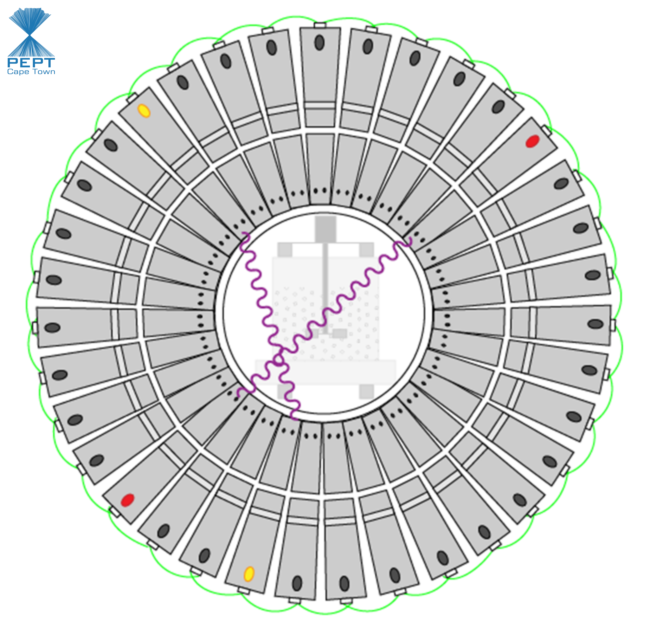
Coincident pairing of gamma rays from a tracer in an experimental vessel.
In principle only two such LORs are necessary, but the recording of non-useful LORs resulting from the detection of gamma rays after undergoing Compton scattering between creation and detection, and the coincident detection of two gamma rays which were not associated with the same annihilation event, mean that a larger number of measured LORs are required. Since many thousands of coincidence events can be processed by a PET camera each second, the location of a moving particle can be tracked at frequencies of up to 1 kHz to produce a series of locations within an uncertainty of the order of magnitude 1 mm.
The precision of the location measurement can be increased by including a larger number of lines in the triangulation of each location. A popular triangulation algorithm is a least squares minimization procedure developed at the University of Birmingham called track. It slices the LOR list into bins of size N and iteratively finds a final fraction f of the closest passing set of lines within some pre-defined limit. These variables f and N can be optimised to minimise the uncertainty in an experiment with a calibration test such as a rotating disc or moving the tracer to fixed points around the vessel. With a series of chronologically recorded LORs, the tracer can be tracked with time to build a trajectory.

Illustration of the triangulation procedure with the Birmingham track algorithm to form a final trajectory.